Neurons & Glia Cells

Fun Fact: Within the compact structure of our brain, there are an estimated trillion neurons, each linking to roughly 10,000 others—creating a staggering total of around 10 million billion connections! Plus, the brain is filled with glial cells, which are estimated to outnumber neurons by about 10 to 1, bringing their total to around 10 trillion.
This page will delve into the fascinating world of the brain, exploring the building blocks of thought, feeling, and action. We'll uncover the intricate workings of neurons and glia, the dynamic process of neurotransmission, and the remarkable ability of the brain to adapt and change through neuroplasticity. We'll also examine the crucial roles of gray matter and white matter in shaping our cognitive abilities. By understanding the structure, function, and diverse roles of neurons and glia cells, we can gain a deeper appreciation for the intricate workings of the brain and its remarkable capacity to shape our thoughts, emotions, and behaviors.
Jump to:
Glia Cells

Often overshadowed by their more renowned counterparts, the neurons, glial cells are the unsung heroes of the nervous system. The name "neuroglia" itself means "nerve glue," highlighting their crucial role in supporting and nurturing neurons. While neurons are responsible for transmitting electrical and chemical signals, glia function mainly to modulate neuron function and signaling, ensuring efficient communication and overall brain health.
Diverse Functions of Glia:
Glial cells are incredibly versatile, performing many diverse functions, including:
​
-
Myelin Production: Some glial cells produce myelin, a fatty substance that wraps around axons (nerve fibers) to speed up nerve impulse transmission.
-
Oligodendrocytes: Produce myelin in the central nervous system (CNS).
-
Schwann cells: Produce myelin in the peripheral nervous system (PNS).
-
-
Synapse Modulation: Glia influence the formation, function, and elimination of synapses, the connections between neurons, thereby shaping the flow of information in the brain.
-
Astrocytes: Play a key role in synapse formation and function in the CNS.
-
-
Blood Flow Regulation: Glia help regulate blood flow in the brain, ensuring that neurons receive the oxygen and nutrients they need to function properly.
-
Astrocytes: Contribute to blood flow regulation in the CNS.
-
-
Metabolic Support: Glia provide metabolic support to neurons, supplying them with energy and essential nutrients.
-
Homeostasis Maintenance: Glia help maintain the proper ionic and water balance in the brain, creating a stable environment for neuronal activity.
-
Astrocytes: Regulate the extracellular environment in the CNS.
-
-
Immune Defense: Some glia act as immune cells in the brain, protecting it from infection and inflammation.
-
Microglia: The primary immune cells of the CNS, they engulf and remove cellular debris and pathogens.
-
-
Cerebrospinal Fluid Production: Some glia produce cerebrospinal fluid (CSF), which cushions and protects the brain and spinal cord.
-
Ependymal cells: Line the ventricles of the brain and the central canal of the spinal cord, producing CSF.
Neurons and Glia: A Dynamic Partnership
​
Neurons and glial cells work together in the nervous system to create the complex behaviors that make us human. Neurons generate and propagate electrical and chemical signals, allowing communication throughout the brain and body. Glia, on the other hand, perform a variety of supportive functions that enable neurons to do their job effectively.
Neurons
Neurons are the fundamental units of the nervous system, the specialized cells that allow your brain to communicate with every part of your body. They are the messengers that carry thoughts, feelings, and instructions, enabling you to experience the world around you and respond to it.
A typical neuron has three main parts:
​
-
Cell Body (Soma): The central part of the neuron, containing the nucleus and other organelles that keep the cell alive and functioning.
-
Dendrites: Branch-like extensions that receive signals from other neurons. They act like antennas, picking up incoming messages.
-
Axon: A long, slender fiber that transmits signals away from the cell body to other neurons, muscles, or glands. It acts like a cable, carrying the message to its destination.
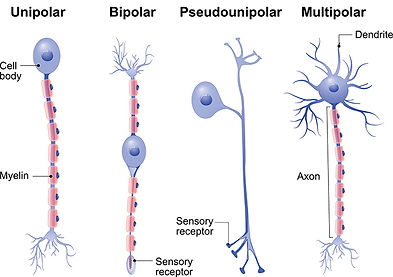
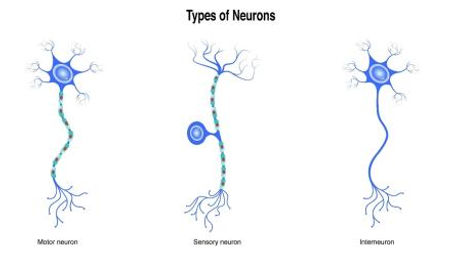
While there are three main categories of neurons, within these categories lie hundreds of different types, each with unique characteristics and abilities to send and receive messages. This incredible diversity contributes to the complexity of the nervous system and the uniqueness of each individual's thoughts, feelings, and actions.
Here are the main types of neurons:
​
-
Sensory Neurons: These neurons are activated by sensory input from the environment, allowing you to perceive the world around you. They respond to physical stimuli like sound, touch, heat, and light, as well as chemical stimuli from taste and smell. Most sensory neurons are pseudounipolar, meaning they have a single axon that splits into two branches, one extending to the periphery (where it receives sensory input) and the other extending to the central nervous system (where it transmits the signal).​
-
Motor Neurons: These neurons connect to muscles, glands, and organs throughout the body, allowing your brain to control movements and bodily functions. They have the most common "body plan" for a nerve cell, with one axon and several dendrites (multipolar). There are two types of motor neurons:
-
Lower Motor Neurons: These neurons extend from the spinal cord to muscles, directly controlling muscle contractions.
-
Upper Motor Neurons: These neurons travel between the brain and spinal cord, relaying signals from the brain to lower motor neurons.
-
-
Interneurons: These neurons connect sensory and motor neurons, forming complex circuits within the brain and spinal cord. They play a crucial role in processing information, generating thoughts, and coordinating responses. Interneurons are also multipolar, with one axon and several dendrites. They can communicate not only with sensory and motor neurons but also with each other, forming intricate networks of varying complexity.​
Neurotransmitters
Imagine your nervous system as a vast communication network, with neurons acting as the individual wires carrying electrical signals. But how do these signals jump the gap between neurons? That's where neurotransmitters come in. These remarkable chemical messengers are the couriers of the nervous system, transmitting information from one neuron to the next, and from neurons to muscles and glands, enabling a wide range of functions, from thinking and feeling to moving and breathing.

There are dozens of different neurotransmitters in the brain, each with specific roles in regulating various functions. Here are some of the most important ones:
-
Acetylcholine: Involved in muscle movement, learning, memory, and attention.
-
Dopamine: Plays a key role in motivation, reward, pleasure, and movement.
-
Serotonin: Regulates mood, sleep, appetite, and aggression.
-
Norepinephrine: Important for alertness, arousal, and the stress response.
-
GABA (Gamma-Aminobutyric Acid): The main inhibitory neurotransmitter in the brain, it helps to calm and relax the nervous system.
-
Glutamate: The main excitatory neurotransmitter, it plays a crucial role in learning and memory.
-
Endorphins: Natural painkillers, they also contribute to feelings of pleasure and well-being.
Neurotransmission
Fun Fact: The synaptic gap is around 20 to 30 nanometers across, a fraction of the size of a human hair, which is approximately 80,000 nanometers in diameter.
Imagine your brain as a bustling city, with billions of neurons acting as the residents, constantly communicating with each other to keep things running smoothly. Neurotransmission is the intricate process by which these neurons send messages back and forth, allowing you to think, feel, move, and experience the world around you.
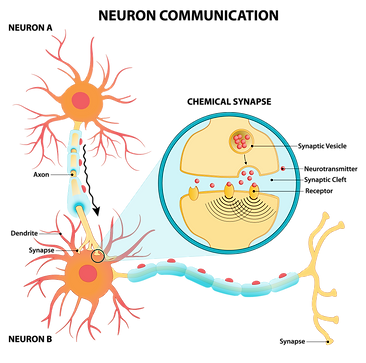

The communication between neurons doesn't happen through direct contact. Instead, there's a tiny gap between them called the synapse. Think of it as a bustling intersection where messages are passed from one neuron to the next.
​
The Steps of Neurotransmission:
​
-
Electrical Signal Arrives: A nerve impulse, or action potential, travels down the axon of the sending neuron (presynaptic neuron), like an electrical current flowing through a wire.
-
Neurotransmitter Release: When the electrical signal reaches the end of the axon (the axon terminal), it triggers the release of chemical messengers called neurotransmitters from tiny sacs called vesicles. These neurotransmitters spill out into the synapse.
-
Crossing the Gap: The neurotransmitters diffuse across the synapse, like tiny boats crossing a river, heading towards the receiving neuron (postsynaptic neuron).
-
Binding to Receptors: On the surface of the receiving neuron's dendrites are specialized proteins called receptors. The neurotransmitters bind to these receptors, like keys fitting into locks.
-
Message Received: This binding triggers changes in the receiving neuron, either exciting it (making it more likely to fire its own electrical signal) or inhibiting it (making it less likely to fire).
-
Signal Termination: To ensure precise control of the message, the neurotransmitters are quickly cleared from the synapse. This can happen through:
-
Reuptake: The sending neuron reabsorbs the neurotransmitters.
-
Enzymatic Degradation: Enzymes break down the neurotransmitters.
-
Diffusion: The neurotransmitters simply drift away from the synapse.
-
Neurons are the master communicators of your nervous system, transmitting information throughout your brain and body. But they don't rely on a single method to get their messages across. Instead, they employ a fascinating combination of electrical and chemical signals, like a sophisticated telegraph system with both wires and coded messages.
​
Electrical Signals: Within the Neuron
Within a single neuron , communication is electrical. Imagine a tiny electrical current flowing down the neuron's axon, like a spark traveling along a wire. This electrical signal, called an action potential, is generated by the movement of charged particles (ions) in and out of the neuron. It's a rapid and efficient way to transmit information over long distances within the neuron.
​
Chemical Signals: Between Neurons
When the electrical signal reaches the end of the neuron, it encounters a gap called the synapse, separating it from the next neuron. To bridge this gap, the neuron switches to a chemical communication system. It releases neurotransmitters, chemical messengers that carry the signal across the synapse. These neurotransmitters bind to receptors on the neighboring neuron, triggering a new electrical signal in that neuron, and thus continuing the message relay.
Think of it this way:
Imagine a relay race. The first runner (the electrical signal) sprints down the track (the axon) carrying the baton (the message). When they reach the end of their leg, they pass the baton (the neurotransmitters) to the next runner (the next neuron) across a short gap (the synapse). The second runner then continues the race with the baton, carrying the message forward.
​
Why Two Signals?
This dual system of electrical and chemical signaling gives the nervous system incredible flexibility and control over information flow. The electrical signals allow for rapid communication within neurons, while the chemical signals provide precise control over the transmission of messages between neurons.
​
This intricate interplay of electrical and chemical signals is the foundation of all brain activity, enabling us to perceive the world, think, feel, and act. It's a testament to the remarkable complexity and efficiency of the nervous system.
Neuroplasticity
Fun fact: Thought alone is associated with neuroplastic gains. Some aging piano performers prepare for concerts primarily through visualization instead of physical practice. Both approaches show the same motor mapping in an fMRI scan.

It was once thought that the brain was a fixed and static organ, its structure and function set in stone after childhood. But groundbreaking research has revealed a remarkable truth: the brain is incredibly dynamic, constantly adapting and rewiring itself throughout life. This phenomenon is known as neuroplasticity, and it's the key to learning, memory, and recovery from injury.
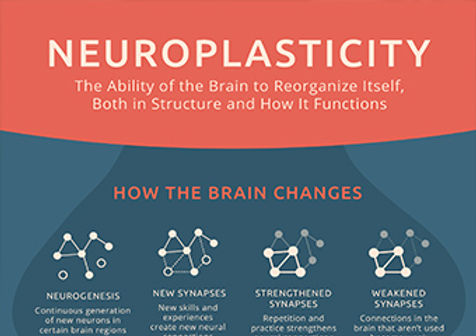
Neuroplasticity, also known as neural plasticity or brain plasticity, is a process that involves adaptive structural and functional changes to the brain. It's the nervous system's ability to change its activity in response to intrinsic or extrinsic stimuli by reorganizing its structure, functions, or connections. These changes occur at multiple levels:
​
-
Chemical: Changes in neurotransmitter release and receptor sensitivity, often associated with short-term memory or skill improvement.
-
Structural: Alterations in the connections between neurons, leading to long-term memory and skill development.
-
Functional: Changes in entire brain networks, making them more excitable and efficient.
​
How Does Neuroplasticity Work?
​
The brain is constantly processing sensory information, and as it does, some connections between neurons (synapses) strengthen while others weaken, depending on which are used. This process, known as synaptic pruning, eliminates inefficient connections, leaving behind only the most effective neural networks.
​​
Types of Neuroplasticity:
​​
-
Developmental Plasticity: Occurs primarily in the first few years of life, involving rapid neurons growth and synapse formation, followed by pruning of unused connections.
-
Homologous Area Adaptation: When one part of the brain is injured during early development, the corresponding region in the opposite hemisphere can take over its function.
-
Compensatory Masquerade: The brain finds alternative ways to accomplish tasks when the usual pathways are damaged, such as after a stroke.
-
Cross-Modal Reassignment: When one sense is lost, the brain can rewire itself to use input from other senses, such as a blind person "seeing" through touch.
-
Map Expansion: The brain area involved in a specific process expands with learning and repetition, like when learning a musical instrument.
Gray Matter & White Matter

Fun Facts:
-
Learning another language increases density in gray matter and strengthens white matter.
-
London taxi cab drivers have greater gray matter volume in mid-posterior hippocampus in comparison to London bus drivers.
While often depicted as a uniformly gray organ, the brain is actually a masterpiece of contrasting colors and textures, composed of two distinct types of tissue: gray matter and white matter. These two components, representing roughly 40% and 60% of the brain respectively, work in perfect harmony to orchestrate the complex symphony of thoughts, actions, and emotions that define our human experience.
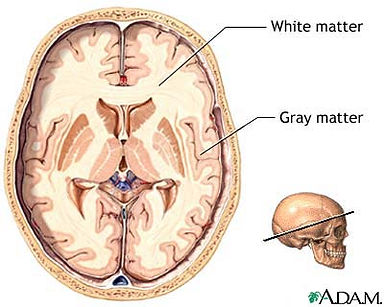
Gray Matter: The Processing Powerhouse
​Gray matter, the darker, outer layer of the brain, earns its name from its characteristic grayish-pink hue. It's a bustling hub of activity, primarily composed of:
​
-
Neuron Cell Bodies: The central part of neurons, containing the nucleus and other organelles that keep the cell alive and functioning.
-
Dendrites: Branch-like extensions of neurons that receive signals from other neurons, like antennas picking up incoming messages.
-
Synapses: The connections between neurons where communication occurs, like bustling intersections where messages are exchanged.
Functions of Gray Matter:
-
Information Processing: Gray matter is where the brain analyzes and interprets information from the senses, making sense of the world around us.
-
Decision Making: It's the seat of our decision-making abilities, weighing options and choosing the best course of action.
-
Memory Formation: Gray matter plays a crucial role in forming new memories and storing them for later retrieval.
-
Higher-Level Thinking: It's involved in complex cognitive functions that make us uniquely human, such as language, attention, reasoning, and consciousness.
White Matter: The Communication Highway
White matter, lying beneath the gray matter, gets its name from its lighter appearance, which is due to the presence of:
-
Axons: The long, slender fibers that extend from neurons cell bodies, transmitting signals to other neurons, muscles, or glands. Think of them as the brain's communication cables, carrying messages near and far.
-
Myelin: A fatty substance that wraps around axons, acting like insulation on electrical wires. Myelin significantly speeds up signal transmission, ensuring efficient communication throughout the brain and body.
​
Functions of White Matter:
-
Communication: White matter acts as the brain's intricate communication network, connecting different regions of gray matter and allowing them to exchange information rapidly and efficiently.
-
Coordination: It facilitates coordinated activity between different brain areas, enabling complex tasks and behaviors, such as walking, talking, and playing a musical instrument.
-
Information Relay: White matter transmits signals between gray matter areas and other parts of the nervous system, including the spinal cord, allowing the brain to communicate with the rest of the body.
Page References
-
https://www.cambridge.org/elt/blog/2022/04/29/learning-language-changes-your-brain/
-
Reisberg, D. (2016). Cognition: Exploring the science of the mind (6th ed.). W. W. Norton & Company.